Have you ever reached for your calipers to take a quick measurement, only to discover the battery is dead? We set out to solve this common frustration in the simplest way possible, while also exploring the latest advancements in small-scale energy harvesting technology along the way.
Takeaways
In this blog, you will learn more about…
- Design time: Energy harvesting requires additional engineering compared to battery-powered systems because you now have a variable energy source and a charge storage system, anticipate more testing for the different use-cases and power mode transitions.
- Component Selection: Low voltage and low quiescent current requirements dramatically reduce the universe of available components.
- Testing Challenges: Test setups are challenging; using the input impedance of devices like the Saleae logic analyzer (1 MΩ) was ~10% of our energy budget per signal.
- Harvesting Efficiency: The lower the power, the lower the harvesting efficiency and there is a hurdle/threshold power level.
This Project: Eco-Calipers
We have many sets of calipers around the office (example1, example2), some that are very low cost and run on a single LR44 battery (also called G13, A76, and L1154). Our solution will work with most of these options.
The CoGS of this design is estimated to be ~$6 per unit at a quantity of 100, with the majority of that cost attributed to the thin film solar cell. LR44 batteries cost about $2.50 each. Based on these figures, this design should pay for itself well within expected lifetime of the calipers compared to continuously replacing batteries. This estimate doesn’t even factor in the added convenience and reduced frustration of never dealing with a dead battery.
What would the breakeven point be if we included the total atmospheric carbon impact or other “true cost” metrics?
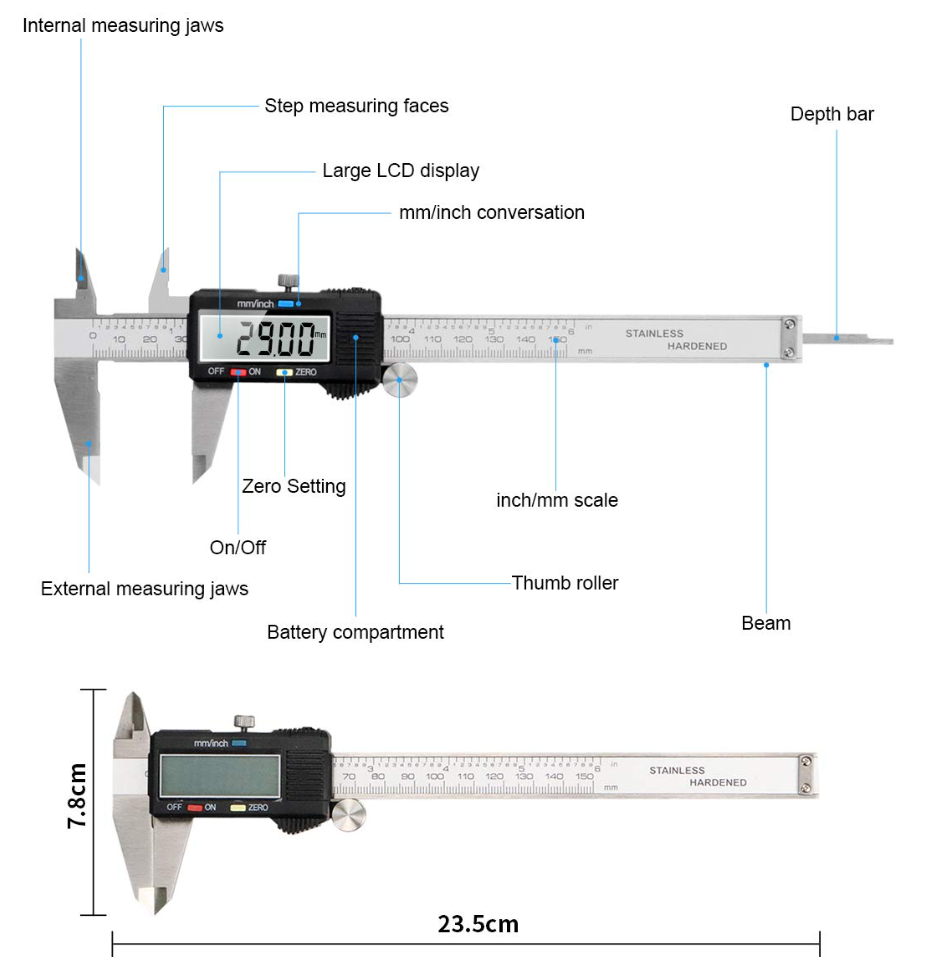
Engineering Design Project 101
This was an internal effort, but we followed 219’s process for new product development as if it were a product we were developing for a client; see sections below. We’ve made good progress and achieved many of our key objectives, reaching roughly 80% completion. We’re pausing temporarily to focus on other project work, but will revisit this and do some of the fine detailed iterations in 2025.
Brainstorm
Goal: To explore unconventional solutions for powering digital calipers.
Requirements
- Instantaneous Operation: The calipers must be ready for use immediately upon removal from the tool chest, with minimal time-effort required to get a single use.
- Environmental Robustness: The calipers must function reliably in a standard office and workshop environment and withstand extended periods of inactivity (months) in a tool chest.
- Mechanical Flexibility: Caliper operation should remain mostly unimpaired even with modifications to the mechanical form factor.
- Target Lifetime: The calipers should be designed for a lifespan of five years with an average use of five times per day.
- Cost and Development Time: We are interested in a significant bit of exploration, but do want to get something functional, perhaps it could accept multiple energy sources as a bonus or alternate.
Ideas
- Kinetic Energy Harvesting: Could we use the caliper’s movement to generate power? This might involve embedding magnets or adding a mass to capture energy during opening and closing. The user would “pump” the caliper before use to charge it.
- Thermal Energy Harvesting: Could we utilize the operator’s hand as a thermal energy source? This assumes the hand temperature is consistently higher than the ambient temperature.
- Inductive Charging Case: A specialized case could house a more substantial energy harvesting solution (e.g., solar panel, larger inductive coil) and recharge the calipers while stored. Spring pins or magnetic coupling could ensure proper alignment and efficient energy transfer.
- Ambient Magnetic Field Harvesting: Could we harvest energy from the building’s 60 Hz magnetic field? This might require a large secondary coil to induce a usable current.
- RF Energy Harvesting: The caliper’s length (6-12 inches) corresponds to a dipole antenna with a resonant frequency of approximately 1.3 GHz ~ 600 MHz. This could potentially capture energy from cell phone towers.
- Solar: The solar calculator seemed an appropriate analog.
Concept Refinement
Goal: Learn about the target device and the energy harvesting solutions.
Teardown & Characterization
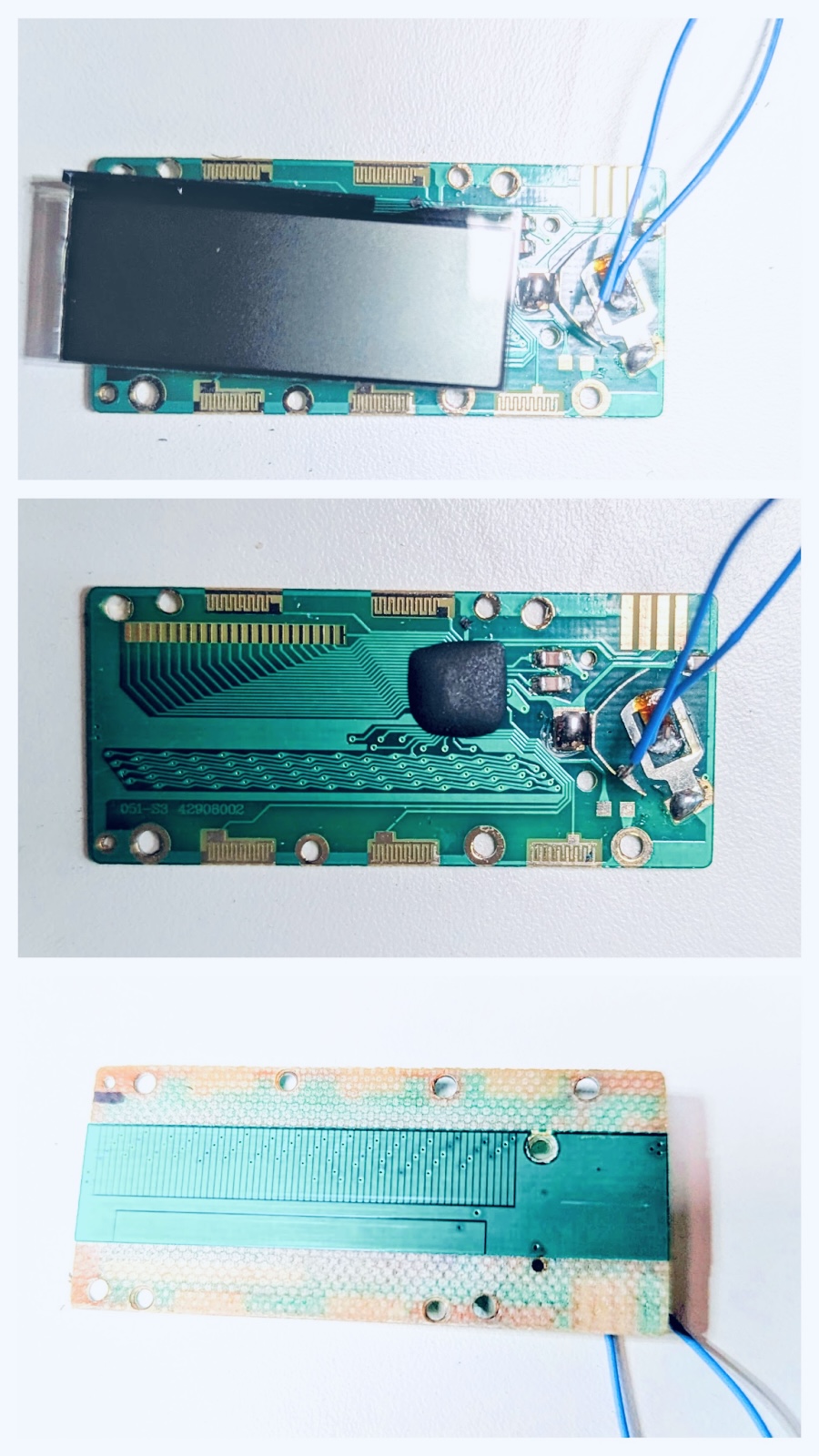
- Battery is a non-rechargeable 1.5V nominal commonly called LR44.
- The relative position measurement involves a phased interleaved reflective capacitance measurement, that is really robust!
- The calipers utilize a chip-on-board (COB) design. which likely incorporates a capacitive switched-mode regulator to boost the battery voltage for LCD operation.
- Zebra connector between LCD and PCB.
- The COB likely incorporates a capacitive switched-mode regulator to boost the battery voltage ~1.5V nominal for LCD operation.
- Bonus: Some caliper models include a data streaming port, which could be helpful for instrumenting these calipers. There are two extra buttons on the PCB that are not exposed in the external housing.
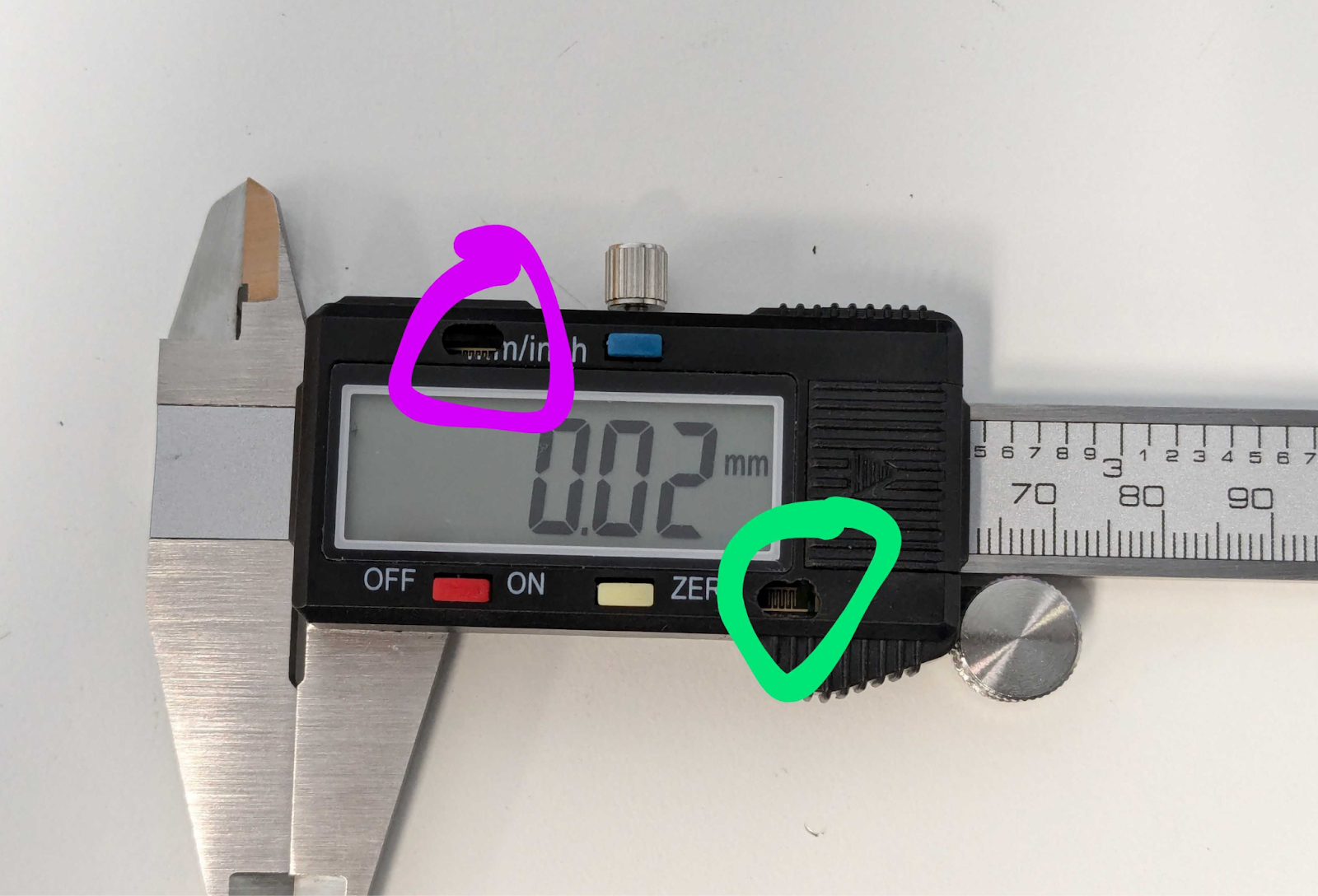
Mechanically we did not want to require modifying the existing calipers assembly, just adding parts. We also want to retain access to the inside jaws, outside jaws and the depth measurement. Our electronics will need to get access to the battery contacts, and we conceived of a battery shaped electrical ‘biscuit’ that would install into the battery compartment, use the battery spring tabs to increase stackup tolerance, and expose the contacts such that it could interface with a flat PCB that is present in the location of the battery door.
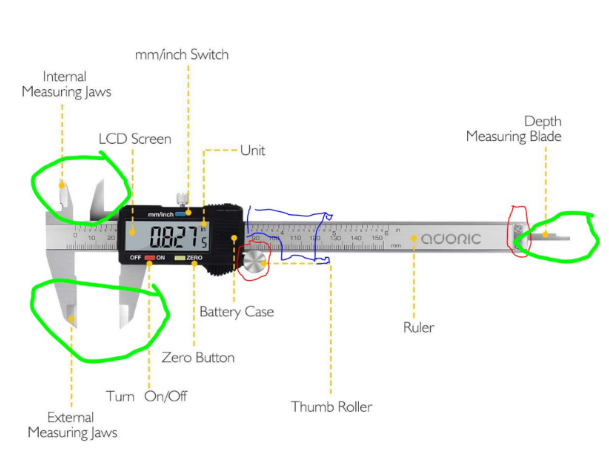
We disassembled three calipers all of which shared the same basic appearance and performance specs, although they did have slightly different design files as evidenced by trivial differences in the PCB and Housing. So it is a mass-produced commodified design that seems pretty optimal in most regards, except for the battery management, as we will see. The calling-cards of this design include: the red, blue and yellow button, 150mm measurement length, 1mil precision, and LR44 battery and sliding battery door.
- When powered on, the calipers consume approximately 23 µW
- Current consumption shows a slight increase as voltage rises, we suspect the capacitor charge pump is not regulated
- Although the display features an auto-off function, the device continues to draw a comparable power of about 20 µW, indicating it doesn’t fully power down
- The calipers function within a voltage range of 1.3V to 1.9V, under 1.3V the device does make proper measurements, over 1.9V the LCD all segments appear on
- LR44 batteries have a capacity of 110~150 mAh and self-discharge rate of ~3% per year, it seems ~20% of capacity may exist below 1.3V. We estimate that the battery should provide about 1 year of shelf life before the calipers are unusable, see the below graph for the battery capacity vs time chart.
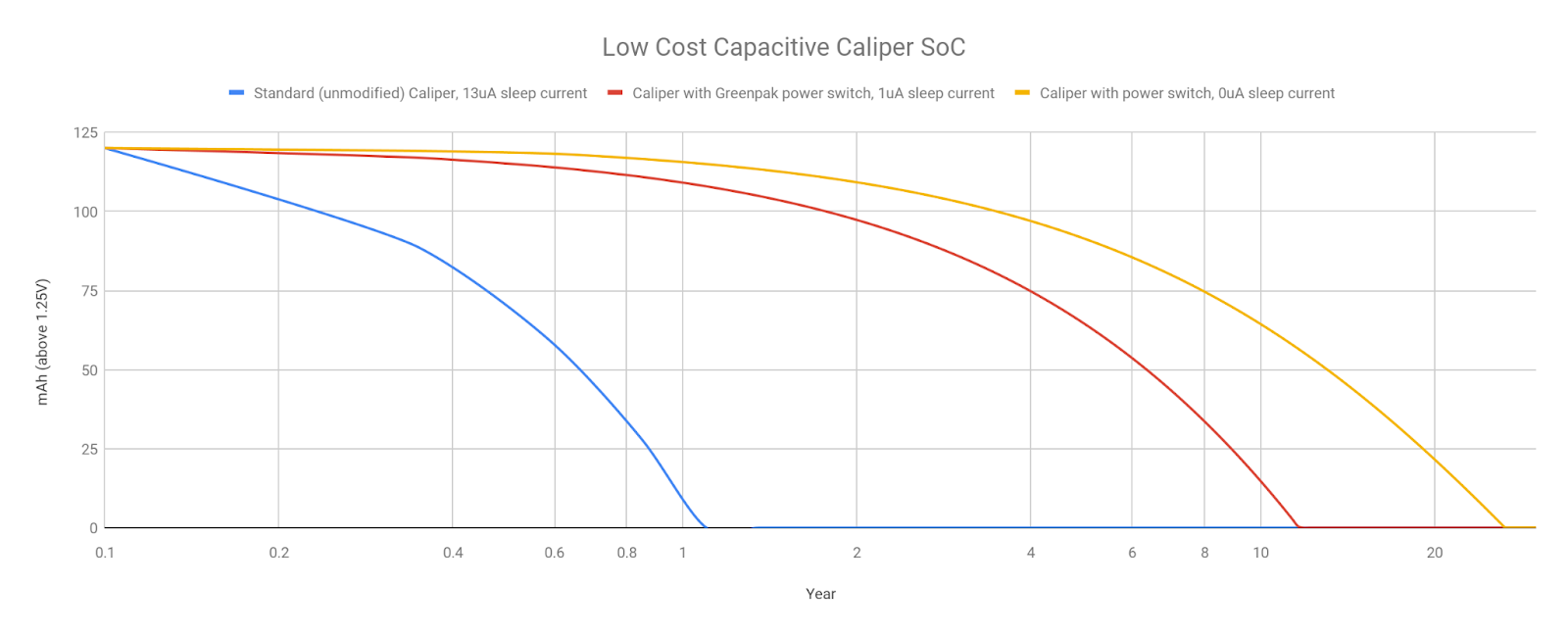
We could theoretically extend the battery life by tenfold just by lowering the sleep current of the calipers via an off switch or a logic chip (e.g. Greepak SLG47512, may draw 1uA and still provide auto-power-off). However we continued the exercise with the goal of getting away from primary batteries. This chart only captures sleep current and self-discharge. We presume an extremely low usage ‘duty cycle,’ the calipers spend most of their life in sleep current.
Energy Storage
We could get 10 minutes of operation from a 2.5V 10 mF EDLC, as it discharges from 2.5V ~ 1.3V with an LDO regulating to 1.5V until the voltage rail collapses. We found some new solid state electrolyte lithium batteries in chip form factor! These have an energy volumetric density that is 10x greater than the standard EDLC (TDK cera charge available for purchase from Digi-Key, and samples from ITEN). These new batteries are safer than the liquid electrolyte and are more tolerant to deep discharge, but do operate at non-standard lithium-ion voltages.
Type | Solution Candidate | Volume (mm3) | Energy (J) | Cost (Qty 1) | Energy Density (mJ/mm3) | Energy Cost Efficiency (mJ/$) | Self-Discharge per Month (% of SoC) |
Electrolytic Capacitor | SVZ0JM102FBRE00REX11 1 mF, 6.3V | 603 | 0.02 | $0.63 | 0.03 | 32 | 99% |
Electric Double Layer Capacitor (EDLC) | FCR0H104ZFTBR24 100 mF, 5.5V | 521 | 1.5 | $5.30 | 2.9 | 285 | 90% |
Lithium Ion Rechargeable with SSE | PWY0250S 250 uAh, 2.7~1.5V | 29 | 1.9 | $13.00 | 66 | 145 | 25% |
NiMH 2s1p | 4545-SB-MC50B-ND 20 mAh, 2.4~1.8 | 720 | 151 | $10.00 | 210 | 15,120 | 20% |
LR44 | LR44 140 mAh, 1.5~1.3V (not rechargeable) | 610 | 706 | $0.21 | 1156 | 3,360,000 | <1% |
SR44 | SR44 160 mAh, 1.5~1.3V (not rechargeable) | 610 | 756 | $3.58 | 1238 | 211,173 | <<1% |
EDLC have ~100x volumetric energy density of electrolytic, Lithium SSE have ~10x the volumetric energy density of EDLC, Alkaline have ~1000x volumetric energy density of Lithium SSE
Energy Source
With power magnitude in mind we did a survey of some of the energy harvesting space, it is a fragmented market that straddles several burgeoning developments, ultra low power, low cost manufacturing, and also various material science disciplines. From this survey we began to see the vast operating regimes of these energy harvesting “transducers.” For context, we believe that a green LED can illuminate for a human detectable pulse with approximately 20 uJ (1mA at 2V for 10 ms) or a continuous 2 mW.
Source | Solution Candidate | Volume (mm3) | Power Avg. (uW) | Cost Qty 1 | Source Impedance | Power Avg. Density (nW/mm3) | Power Cost Efficiency (uW/$) | Comment |
Ambient RF | EP112 10 x 2.3 x 2.3 mm (requires clearance) | 336 | 1 | $5.00 | high | 3 | 0.2 | estimate, 50% energy conversion efficiency |
Piezo | S118-J1SS-1808YB 55 x 23 mm | 593 | 49 | $25.27 | high | 83 | 3 | Quoted peak power at 1% duty cycle, 70% energy conversion efficiency |
Kinetic Switch | ECO 260 30 x 20 x 7 mm | 3999 | 15 | $6.88 | low | 4 | 3 | Quoted peak power at 10% duty cycle, 70% energy conversion efficiency |
TEG | TEG (generic) 40 x 40 mm | 2970 | 110 | $4.98 | low | 37 | 22 | With 30C temp delta, 70% energy conversion efficiency |
Solar | BCSC452B3 25 x 19 mm | 71 | 26 | $4.05 | diode | 360 | 6 | 200 lux, 90% energy conversion efficiency |
Piezo power can be very peaky with frequency, Ambient RF is exciting but challenging, and Solar voltage can be adjusted by selected panel series configuration
Energy Management
We then explored some of the power management chips. The goal of these chips:
- Operate your energy source at MPP (max power point)
- Store any excess power into a storage element and keep it within normal limits, related to charge/discharge voltage/current
- Deliver a regulated voltage for your load
- Handle power-up and power-down transitions
- Extremely low power consumption
These were some of the leading integrated solutions we identified for the two broad classes or energy transducers, low voltage and high voltage:
Part | Output | Features | Application | EVM |
ADP5091 | Adjustable LDO, unregulated Sys | Adjustable Battery Thresholds | Low voltage, continuous sources (TEG & Solar) | EVAL-ADP5091 |
AEM10941-QFN | Adjustable LDO x 2, Adjustable Buck | High integration, works from ~50 mV to 5 V sources | Low voltage, continuous sources (TEG & Solar) | EVK10941 |
SPV1050 | LDO x 2 | 550 mV and 30 μA to Cold start or 2.6V and 5 uA in buck-boost mode | Low voltage, continuous sources (TEG & Solar) | STEVAL-ISV019V1 |
LTC3588 | Adjustable buck | Built-in rectifier, Over Voltage Protection (OVP) | AC or transient, higher voltage (Piezo, Dynamo) | LTC3588 – SparkFun |
There are two broad types of PMU controllers, one for continuous energy sources and one for transient sources.
PMU parts for energy harvesting tend to have slightly different operating voltages for their energy sources, energy storage elements, and loads, as well as varying operating modes for charge transfer and regulation. Selecting the optimal solution requires a detailed evaluation of quiescent current in different modes, cold-start and keep-alive voltages, and energy and voltage thresholds for different mode transitions.
We selected the ADP5901 from Analog Devices. Perhaps we should have also looked to see active datasheet updates, as a proxy for excellent adoption and support. It was still at revision A and had a few obvious errors.
Key Component Evaluation
We purchased some EVMs and did some testing. From this we selected solar photovoltaic to power the calipers for power density and ease of use. We are going to use amorphous solar cells of three series cells. A discretely implemented bang-bang regulator and an EDLC for our storage.
We also tested other regulators, ideal diodes and load switches. Below are some of the detailed findings.
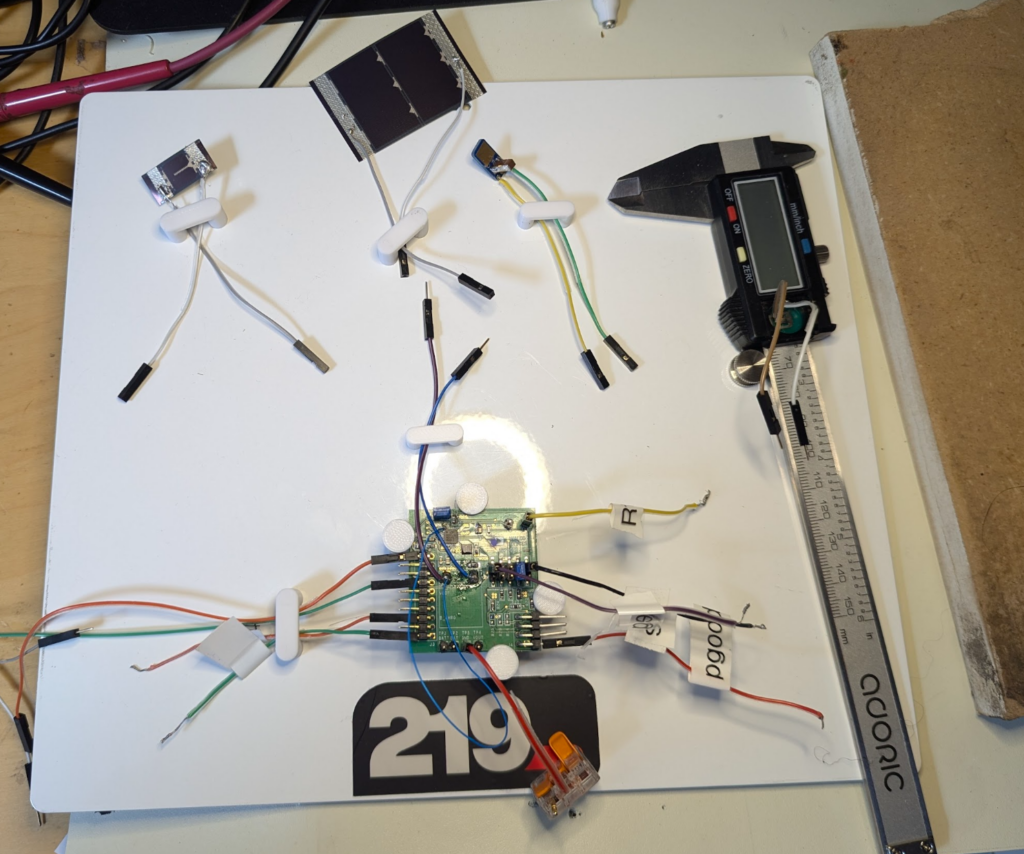
PMU Testing
- The candidate power management unit (PMU) ADP5091 required that our storage element reach a certain voltage before it would deliver a regulated output voltage with the efficient synchronous operating mode, so there was a symmetry between how long it took to charge up and how long it would work without more power. The unregulated output called sys, which is the first cache of charge this chip stores, was insufficient to operate our calipers. This was not ideal as we wanted it to power the load immediately.
- The part had a hard time transitioning from asynchronous boost to synchronous boost with our minuscule power sources. This was a problem because we expected that the device would frequently lose all charge and thus need to undergo a ‘cold start.’
- We were able to select a solar cell whose MPP matched our devices, and since we wanted to operate on streaming energy rather than accumulated energy, this meant an integrated PMU was not the right solution for this project.
Solar Testing
- We evaluated both amorphous and monocrystalline solar cells but amorphous worked better for our low light application. Monocrystalline solar cells are more efficient but only at much higher light levels. Note that amorphous solar cells that are continually used outdoors experience an accelerated degradation. Our SF workshop is about 300 lux with the overhead lights on and 150 lux with the lights off.
- Three quick facts about solar cells in their typical operating mode:
- Solar cell current is linear to irradiance
- Solar cell open circuit voltage is linear with the log of irradiance
- Solar cells MPP is at the knee of the current vs voltage plot and the area contained within is called the fill factor comprises several efficiency factors: series resistance, shunt resistance, and recombination losses within the cell
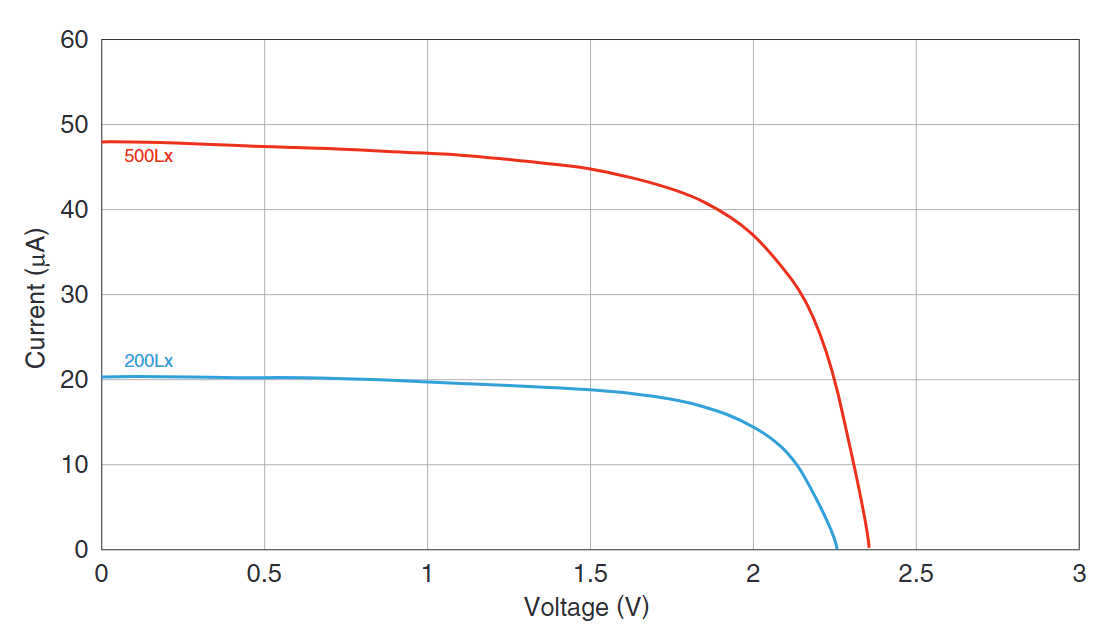
The values at the y-axis intercept 77 uA / 500 lux = 32 uA / 200 lux
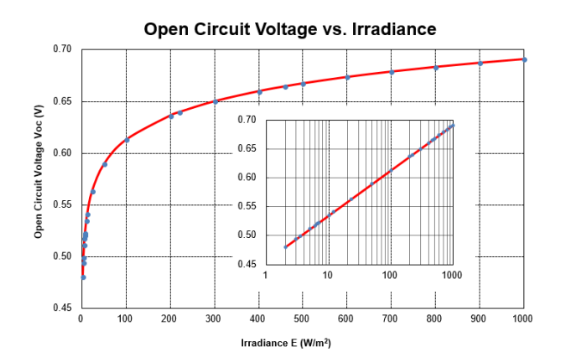
Open circuit voltage is linear with the log of irradiance
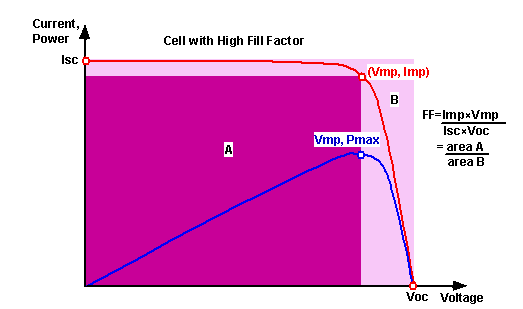
Fill factor is the ratio of the maximum power output to the theoretical maximum power, calculated as the product of the short-circuit current (Isc) and the open-circuit voltage (Voc), it is impacted by series and shunt resistance of the cell (graphic source)
Mechanical Testing
- Hanging our electronics off of the battery door plastics was too tenuous so we mounted it to the caliper beam.
- Our ‘battery biscuit’ had a little difficulty making reliable connections so we installed a spring pin and we found a washer that worked well for the outer electrode.
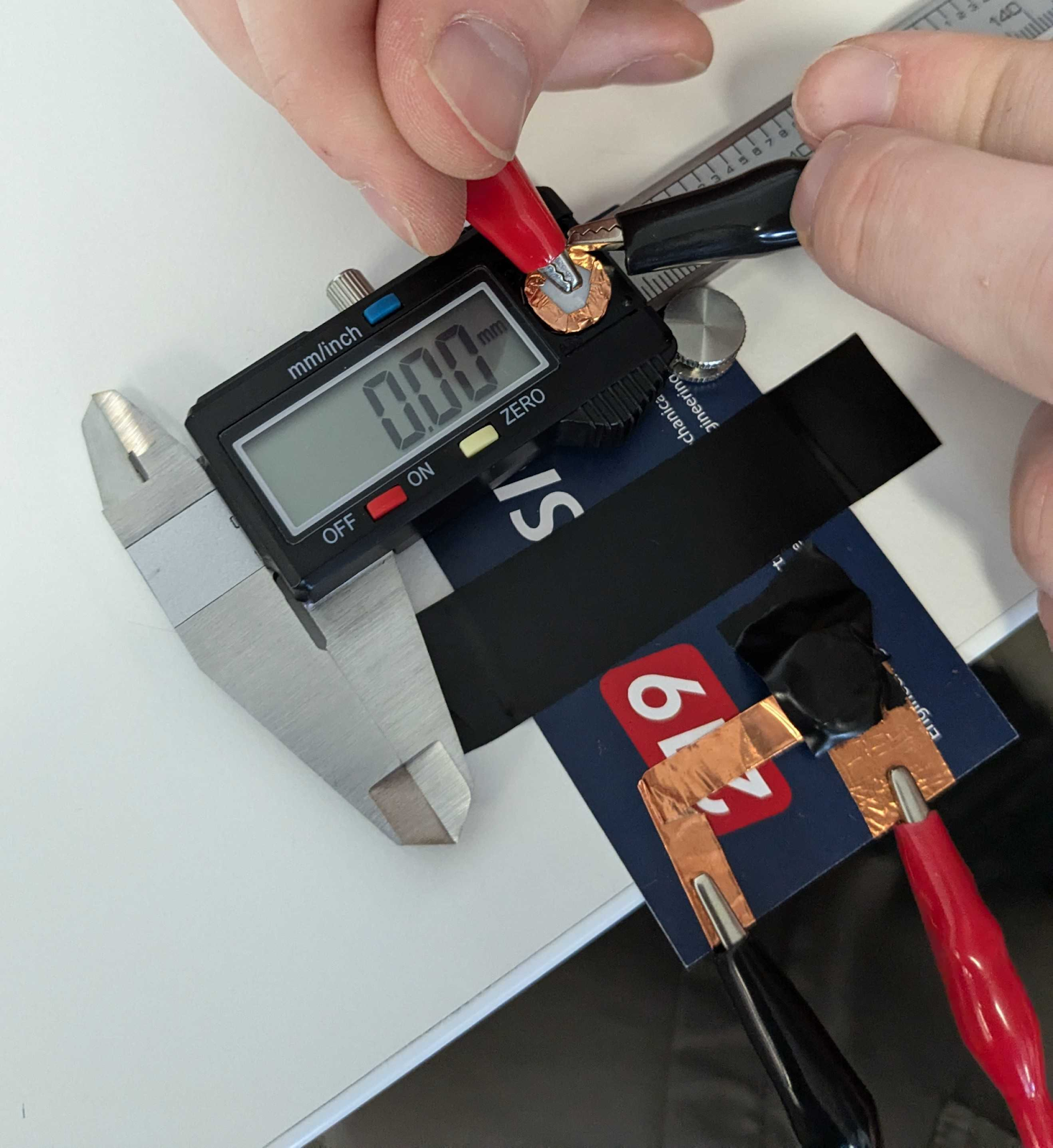
Detailed Engineering
Storage
We aim to immediately power the calipers upon illumination first filling a small ceramic capacitor and then, if any excess charge generated by the solar panel accumulates it in a larger storage EDLC capacitor. We implemented a discrete bang-bang (hysteretic buck) utilizing a window comparator part with a built-in reference (MIC833YM5-TR). Load switches are implemented discretely with an RC circuit and mosfets allow adjustable dead time and thresholds.
This approach ensures that we have enough short-term energy storage to power the calipers for a few seconds, even under fluctuating light conditions. A 10 mF EDLC (CPH3225A-2K) serves as the deep storage element.
Source
Matched our energy source voltage and power knee with our load with the small amorphous solar cell (AM-1456CA-DGK-E). We found a Schottky diode with a very low Vf ~150 mV drop at 15 uA (BAT6302VH6327XTSA1).
Power Management
UVLO (Under-Voltage Lockout): We oversized our solar panel, such that UV is much less likely to occur and this was in part because we had a hard time sourcing an UVLO. In the event of an UV issue, where the calipers enter a weird twilight state, we added a tiny switch to fully cut power in order to reboot. This is preferred over having to hold your hand over the panel for ~10 secs.
OVP (Over-Voltage Protection): We found a low quiescent current low dropout linear regulator that could give us 1.5V output, which would keep the voltage seen by the caliper within its recommended range (STLQ015M12R).
Mechanical
We beveled the top and bottom of the biscuit to improve the reliability of the electrical connections. We had to create a ramp in the circuit board to prevent the spring pin in the battery biscuit from acting as a latch preventing disassembly. Iterating on such small parts is fantastically fast, especially with a core-xy printer.
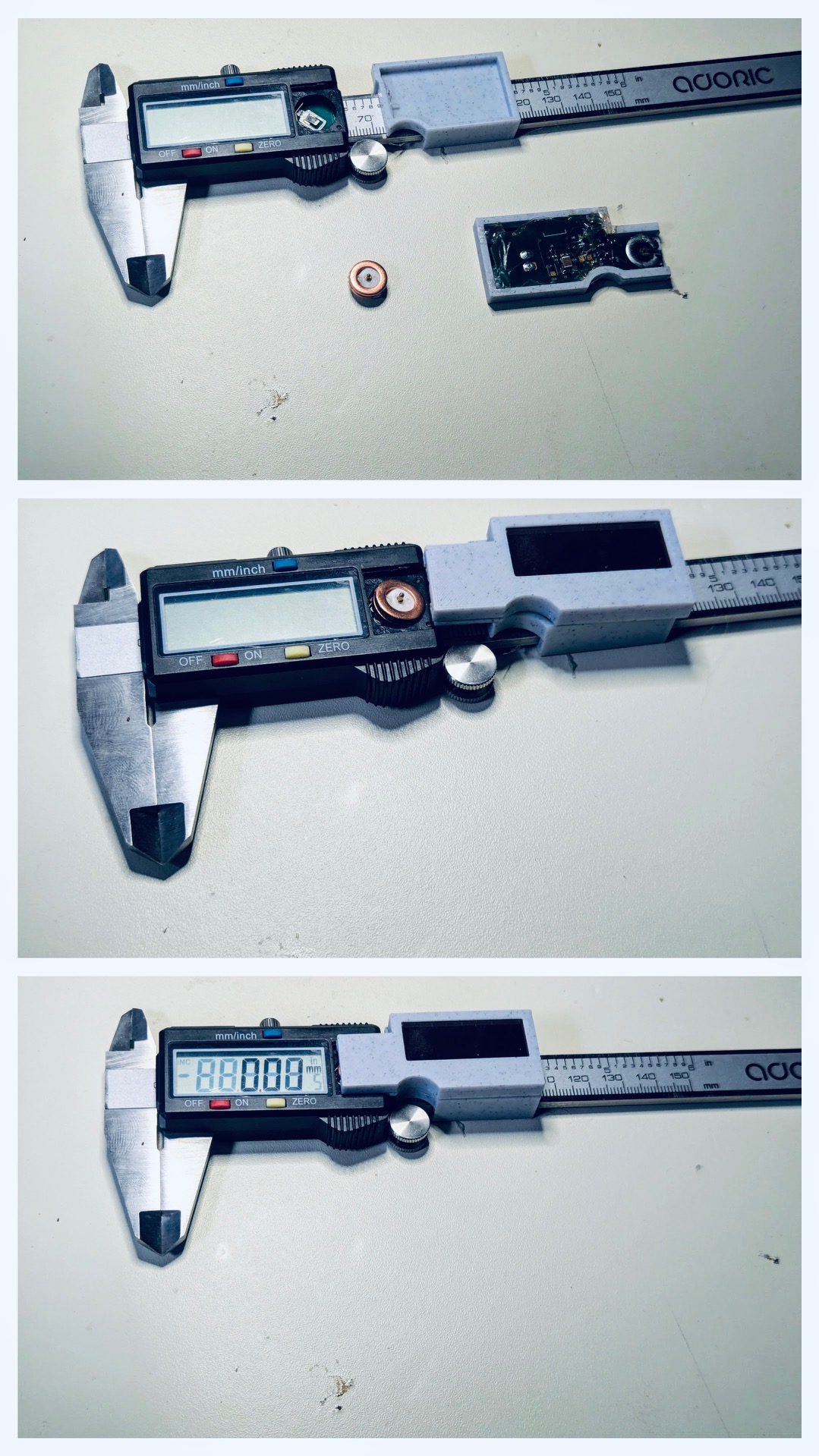
Applications
Just a few examples of products that do or could exist using present-day energy harvesting technologies:
Cordless Devices:
- Keyboards
- Smart Cards
- Remote Controls
Infrastructure:
- Lumineers
- Electronic Labels
- Home Automation Sensors
- Industrial Monitoring Sensors
Tracking Devices:
- Livestock Trackers
- Shipment Trackers
Vehicles:
- Tire Pressure Monitoring Systems (TPMS)
Wearable Devices:
- UV Light Exposure Sensors
- Thermoelectric Generator (TEG) E-Ink Smartwatches
- Rolex Oyster Perpetual Watch (mechanical watch that harvests energy from wrist movement)
Industry
A few organizations focused on the energy harvesting space.
Chip Makers & Integrated Solutions:
- Analog Devices: Several energy harvesting relevant parts going back a dozen years in their portfolio.
- Ambiq: Known for its ultra-low-power MCUs. While not solely focused on energy harvesting, Ambiq’s MCUs are crucial in these systems as they minimize energy consumption, allowing devices to operate for extended periods on harvested energy.
- EnerHarv: An industry conference or workshop focused on energy harvesting technologies. It likely brings together researchers, engineers, and companies to discuss the latest advancements and challenges in the field.
- Enocean: Specializes in energy harvesting wireless modules, particularly known for their self-powered switches and sensors. They use various energy harvesting methods like mechanical (button presses), solar, and thermal. Enocean modules are commonly found in building automation, industrial controls, and smart home applications.
- Epeas: A leading PMU developer with a strong focus on energy harvesting. They offer PMICs designed to efficiently extract power from various sources like solar, vibration, and thermal gradients. Epeas stands out for providing excellent evaluation modules (EVMs), comprehensive datasheets, and helpful design tools, making it easier for engineers to integrate their solutions.
- ITEN: New solid state electrolyte battery manufacturing sampling product now.
- Mide Technology: Experts in vibration energy harvesting. They offer a range of piezoelectric vibration energy harvesters and complete energy harvesting solutions for condition monitoring, wireless sensors, and industrial applications.
- Nordic: Ultra low power wireless SoC and great developer support.
- ONiO: This startup aims to be a one-stop shop for energy harvesting solutions. They are developing a highly integrated chip that combines a power management unit (PMU), a microcontroller (MCU), and a radio transceiver. This “all-in-one” approach simplifies design, reduces the number of external components (passives), and lowers the overall Bill of Materials (BOM) cost. This is ideal for compact, low-power IoT devices.
- Powercast: This company focuses on radio frequency (RF) energy harvesting. They develop technology that captures RF energy from ambient sources (like radio waves) or dedicated transmitters and converts it into usable power. This enables battery-less or wirelessly powered devices.
- Renesas: GreenPak products are low power, low cost and highly configurable
Texas Instruments: a wide range of components relevant to energy harvesting, including PMUs, sensors, and low power SoC wireless chips.
Loose Ends
All of the performance figures are lacking context and utilize simplifying assumptions. It is a fascinating and challenging space. If you are looking to explore energy harvesting for your device, please contact us at getstarted@219design.com.